Speeding up research: developing new technologies to help bring research to life
Enzymes are nature鈥檚 catalysts, they make life possible. Their catalytic power is the basis of the current biotechnology revolution; yet, our understanding of enzymes remains limited by their intrinsic complexity.
The in the at The University of Manchester is developing new technologies to understand how these amazing natural catalysts work with the overall goal of applying this knowledge to address two urgent challenges: first, obtaining a better understanding of how enzymes go wrong in human disease and how to correct them, and secondly, finding more predictable ways to engineer new enzymes for industrial use.
Enzymology in the genomics age
When the human genome was first sequenced in 2003, it ushered in what is now colloquially known as the 鈥済enomics age鈥. Since this monumental achievement, the technologies for sequencing DNA have grown exponentially, culminating in our current ability to rapidly and cheaply sequence DNA. We now possess the ability to routinely sequence patient samples, presenting numerous possibilities for 鈥減recision medicine鈥, where therapies could be tailored to correct disease-causing mutations in enzymes at the individual patient level.
However, this presents a challenge in that there is a wide range of variation in DNA sequences and not all of these variations cause disease; thus patient sequencing efforts often identify hundreds of genetic variations (variants of uncertain significance or VUS), but only some of which actually cause disease. A second challenge is that because the DNA provides the instructions to make the enzymes, the DNA sequence tell us little about how a particular mutation might compromise the actual function of the enzyme itself. The critical step is, therefore, to determine whether a particular VUS disrupts the enzyme鈥檚 function (thus contributing to disease) and is producing the mutated enzyme, and then measuring its biochemical effects: for example, a particular mutant could slow the enzyme down too much, change its reaction, or simply signal to the cell to remove it or direct it to the wrong place, among other potential effects.
A new technology to rapidly measure functional variations
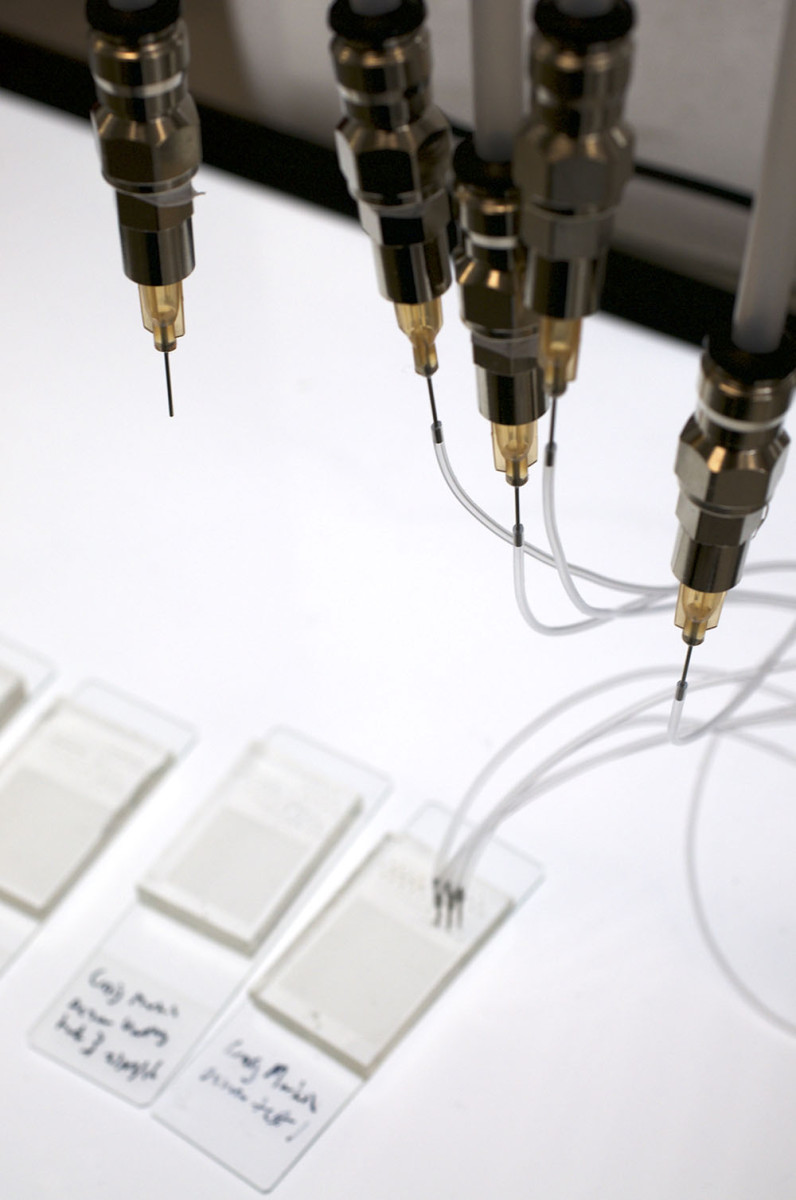
This is what we study in the Markin Lab; the enzyme-level effects of the DNA sequences. Unlike DNA sequencing, the technologies that form the basis of biochemistry and enzymology (the biochemical study of enzymes) have lagged in terms of throughput (that is, the number of measurements that can be made in a given timeframe), accessibility, and widespread adoption. Enzymes are still typically studied 鈥渙ne-at-a-time鈥 using methods largely unchanged for decades and as such it is not possible to rapidly and cheaply biochemically profile VUS using traditional biochemical methods. To address this, the Markin Lab uses a technology called HT-MEK (High-Throughput Microfluidic Enzyme Kinetics (HT-MEK)). Dr Markin developed the HT-MEK technological platform at Stanford University and has since developed it through his work at 91直播.
The central technological innovation underlying HT-MEK is a microfluidic chip roughly the same size as a postage stamp in which 1,500 different enzyme variants can be simultaneously produced, purified, and quantitatively measured with the same (or even higher) accuracy as traditional methods. HT-MEK thus allows thousands of VUS to be functionally profiled in a similar timeframe (1-2 weeks), and at similar cost (< 拢5 per variant),as currently required for a handful of variants using traditional methods.
Enzymes are at the heart of over 7,000 years of biotechnology
Beyond the role of enzymes in human health, humans have harnessed the catalytic power of natural enzymes from other organisms to improve our quality of life for thousands of years. For example, brewing and baking (which rely on the enzymes required for yeast metabolism) originated around 7,000 years ago 鈥 even before achievements such as coinage and written language.
Since these initial biotechnological discoveries, our relationship with and dependence on enzymes has grown into a multi-billion-pound industry. In the Industrial Revolution, brewing and baking become industrialised, representing a key step towards the modern biotechnology industry. In the modern world, we now encounter enzymes frequently in daily life, often without realising it. Applications range from the mundane 鈥 for example, enzymes are used as detergent additives, stain removers, and sink de-cloggers 鈥 to the highly specialised production of therapeutics and fine commodity chemicals. Most recently, enzymes have received special interest due to the potential of harnessing their catalytic power as part of new carbon capture technologies as well as in bioremediation 鈥 a popular example being the degradation of plastic waste.
Millions of years of natural evolution coupled with the broad diversity of life on this planet has provided a wealth of different enzyme catalysts, many thousands of which having been sequenced at the DNA level. These enzymes almost certainly catalyse novel reactions and could help fuel these next-generation biotechnology applications; however, like the VUS variants described above, the current challenge is that their actual functions (i.e. what molecules they make and how fast they can make them) are difficult to predict, and the vast majority have not yet been characterised. The Markin Lab, in collaboration with expert research groups in the 91直播 Institute of Biotechnology, has begun to now expand HT-MEK to measure the function of hundreds to thousands of these enzymes simultaneously. In this way, we can more rapidly (and more cheaply) identify new promising catalysts for a diversity of applications.
Open and accessible technologies to fuel the 鈥渆nzyme age鈥
Drawing comparison again to the development and adoption of DNA sequencing technologies, the ultimate success (and potential impact) of this approach hinges on providing the wider biochemistry and enzymology community with the capability to carry out similar high-throughput experiments in their own labs. To this end, the Markin Lab is also developing new open-source hardware and software to fabricate and run HT-MEK microfluidic chips at a fraction of the original cost, as well as developing new measurement modalities to apply high-throughput approaches to a wide cross-section of enzymes. The ultimate vision is to usher in a new age of quantitative biochemistry where these technologies are accessible and available to all research groups, and where quantitative studies of thousands of enzymes are as routine as studies of single enzymes are currently.
Words and pictures - Dr Craig Markin